1 The origin of STEM education
In this chapter you will learn about:
- What is the origin of STEM education?
- How STEM education is interpreted?
Driven by current and future shortages in the STEM workforce, many education systems and policy makers around the globe are preoccupied with advancing competencies in STEM domains, but what does the STEM stand for? The acronym STEM was coined in 2001 by Judith A. Ramaley of the National Science Foundation (USA) and replaced the previously used acronym SMET (Sanders, 2009). Both acronyms initially appeared to serve the sole purpose of referring to the Science, Math, Technology and Engineering disciplines and the professionals working in them. NSF has later composed a list of STEM fields, including the industry-relevant areas of psychology, social sciences, and education and learning research.
STEM now is used as a brand name to describe the integration of science, technology, engineering, and mathematics in educational curricula. J. Ramaley defined STEM as an educational inquiry where learning was placed in context and students solved real-world problems through creation of opportunities—a pursuit of innovation (Chute, 2009). Her concept provided an answer to the relatively poor performance of American students in standardized exams for mathematics and science as well as the need to bolster the declining enrollments in universities in the academic STEM fields (Dugger, 2010).
Talking about the integrational models of Science, Technology, Engineering and Mathematics, the currently used definitions are:
S — Science, which deals with and seeks the understanding of the natural world (NRC, 1996, p. 24), is the underpinning of technology. Science is concerned with what is (exists) in the natural world. Some of the processes that are used in science to seek out the meaning of the natural world are “inquiry,” “discovering what is,” “exploring,” and using “the scientific method.”
T — Technology, on the other hand, is the modification of the natural world to meet human wants and needs (ITEA, 2000, p. 7), or “The goal of technology is to make modifications in the world to meet human needs” (NRC, 1996, p. 24). Technology is concerned with what can be (designed, made, and developed) from natural world materials and substances to satisfy human needs and desires. Some processes used in technology to alter and change the natural world are “invention,” “innovation,” “practical problem solving,” and “design.”
E — “Engineering is the profession in which a knowledge of the mathematical and natural sciences gained by study, experience, and practice is applied with judgment to develop ways to economically utilize the materials and forces of nature for the benefit of mankind” (ABET, 2002). There are strong philosophical connections between the disciplines of technology and engineering.
M — “Mathematics is the science of patterns and relationships” (AAAS, 1993, p. 23). It provides an exact language for technology, science, and engineering. Developments in technology, such as the computer, stimulate mathematics, just as developments in mathematics often enhance innovations in technology. One example of this is a mathematical model that can help the technological design by simulating how a proposed system may operate.
The above-mentioned disciplines and approaches of learning about the surrounding world can be merged or interact in several models, a few are depicted in Fig. 1.
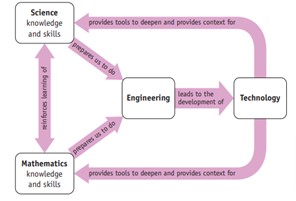
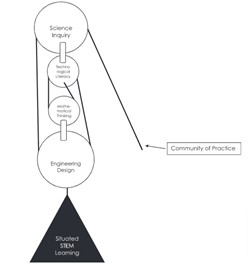
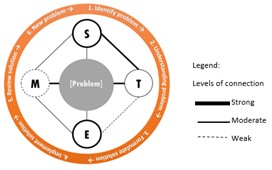
Fig. 1. The subject relationship models of STEM. A. Minnesota STEM initiative (cit.). B. Kelley and Knowles, 2016. C. meriSTEM, Singapore (Tan et al., 2019).
As an example we can take a look at the activity “Micro Gardening”. This activity focuses on the importance of water such as water as a means of transporting nutrients and the scarcity of drinking water. If you compare growing crops in hydro-culture to growing in soil, less water is used growing in hydro-culture.
In this activity STEM is integrated

- S: investigating under what conditions plants grow, comparing them with open-air conditions and soil.
- T: set up a test rig with plants, hydroculture, artificial light.
- E: enhance growth by using the right parameters
- A: designing an inside garden, prototyping (both functional and aesthetic.
- M: convert growing conditions data into a graph
STEM education can link scientific inquiry, by formulating questions answered through investigation, to inform the student before they engage in the engineering design process to solve problems (Kennedy and Odell, 2014). Rosicka (2016) advocates that the STEM approach should increase students’ motivation, improve problem-solving skills and achievements in mathematics and science, thereby helping students to understand not only what they are learning, but also where it can be applied.
Quality STEM education could sustain or increase the STEM pipeline of individuals preparing for careers in these fields (Stohlmann et al. 2012). Improving STEM education may also increase the literacy of all people across the population in technological and scientific areas (NRC 2009; 2011).
Definitions of STEM education:
Although a number of countries, including the majority of the EU members, have national strategies concerning STEM education, there is no internationally approved definition. Among the mostly used approaches are:
- Teaching and learning between/among any two or more of the STEM subject areas, and/or between a STEM subject and one or more other school subjects (Sanders, 2009);
- Effort to combine some or all of the four disciplines of science, technology, engineering, and mathematics into one class, unit, or lesson that is based on connections between the subjects and real-world problems. Integrated STEM curriculum models can contain STEM content learning objectives primarily focused on one subject, but contexts can come from other STEM subjects (Moore et al. 2014);
- Approach to teaching the STEM content of two or more STEM domains, bound by STEM practices within an authentic context for the purpose of connecting these subjects to enhance student learning. Practices should include science inquiry, engineering design, mathematical thinking and technological literacy (Kelley and Knowles, 2016).
Despite the disputes of how many disciplines should be included, STEM activities involve them in complex projects that require the application of skills and knowledge from all of them at once, which is closer to how they will one day use their abilities in their everyday life.
Project based learning is the dominant approach in STEM education. For example, students may learn geometry, physics, and biology to build a working greenhouse or include automatization to integrate the learning of IT. These projects, however, tend to take a purely functional approach to the task and utilitaristic interdependence of the subjects.
On occasion the projects may incorporate design elements to combine function and form, however, the more in-depth integration of human (or social)-oriented aspects of the “product“ of STEM projects was implemented upon the transition to the STEAM. Following the direction of more in-depth integration, we are meeting the phenomenon-based learning, which is a learner-centered, multidisciplinary instructional approach that is based on student inquiry and problem solving without focusing on teaching specific subjects, nor is there any preset learning objective. Instead, learners investigate and solve their own questions by applying what subjects are relevant to the problem (Valamis, 2019). In STEM and STEAM, however, the central role is placed on the S-T-E-M subjects, enriching with connections to other disciplines.
References
American Association for the Advancement of Science. (1993). Benchmarks for science literacy. Oxford University Press.
Chute, E. (2009). STEM education is branching out: Focus shifts from making science, math accessible to more than just brightest. Pittsburg Post-Gazette, 947944–947298.
Dugger, W. E. (2010, December). Evolution of STEM in the United States. In the 6th Biennial International Conference on Technology Education Research’nda sunulmuş bildiri, Gold Coast, Queensland, Australia.
ITEA. (2000/2/2007). Standards for technological literacy: Content for the study of technology. Reston, VA.
Kelley, T. R., & Knowles, J. G. (2016). A conceptual framework for integrated STEM education. International Journal of STEM Education, 3(1), 11. https://doi.org/10.1186/s40594-016-0046-z
Kennedy, T., & Odell, M. (2014). Engaging students in STEM education. Science Education International, 25(3), 246–258.
Moore, T., Stohlmann, M., Wang, H., Tank, K., Glancy, A., & Roehrig, G. (2014). Implementation and integration of engineering in K-12 STEM education. In S. Purzer, J. Strobel & M. Cardella (Eds.), Engineering in pre–college settings: Synthesizing research, policy, and practices (pp. 35–60). Purdue University Press.
National Research Council. (1996). The national science education standards. National Academy Press.
Rosicka, C. (2016). Translating STEM education research into practice.
Sanders, M. (2009). Integrative STEM education: Primer. Technology Teacher, 68(4), 20–26.
Stohlmann, M., Moore, T., & Roehrig, G. (2012). Considerations for teaching integrated STEM education. Journal of Pre-College Engineering Education Research, 2(1), 28–34. https://doi.org/10.5703/1288284314653
Tan, A. L., Teo, T. W., Choy, B. H., & Ong, Y. S. (2019). The STEM quartet. Innovation and Education, 1(1), 1–14.
Valamis. (April 19, 2019). Phenomenon-based learning. https://www.valamis.com/hub/phenomenon-based-learning, Retrieved [2021/4/19]